Veins and tracheae: formation of filament-like branching networks
Filamentous branching structures are a common pattern element in all higher organisms. They are used to supply the tissue with nutrition, water, oxygen and information and drain the tissue. The venation of leaves, the tracheae of insects, the blood or lymph vessels as well as neurons are examples. How can such complex patterns emerge?
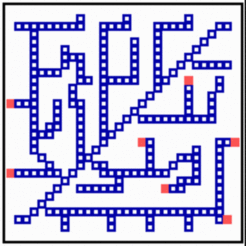
According to the model proposed, filaments appear like traces behind local signals that wander over the field. In the simulation above, it is assumed that local signals are generated by an activator (red) - inhibitor system. When these signals are above a certain threshold, the exposed cells to differentiate into members of the filament system. Differentiated cells repel the signal, causing in this way their shift into a neighboring region. These cells also differentiate and the filament becomes elongated, and so on. To orient the elongation into a region not supplied by filaments, all cells are supposed to produce a substance (green) that is removed by the differentiated cells. The substrate concentration is a measure of how urgently the cells need the in growth of veins, for instance, in order to remove an oxygen deficiency. Due to the activator-inhibitor mechanism, there is a competition along the filament and those regions will win and become elongated the that are exposed to the highest substrate concentration. This is usually the tip region of a filament. A repetition of this process - differentiation, shift of the signal, differentiation - leads to a long strand of differentiated cells behind a wandering activator maximum (Meinhardt, 1976, 1982, 1998; the essence of the model is also available in a commented PowerPoint presentation [PPT]). For tracheae in insects, most of the predicted ingredients have been found (see [1,2]. The addition of newly differentiated cells is one possible realization. In nerves and tracheae, for instance, the elongation of the filaments occurs essentially by the local elongation of individual cells.
Branches are formed whenever activator maxima become sufficiently remote from each other during elongation of filaments. Then, the inhibitor concentration can become locally so low that a new activator maximum is triggered along an existing filament due to a small baseline activator production of the differentiated cells. Whether a branch is formed towards the one side or the other depends on minute fluctuations. However, if a branch has been formed, let us say, to the right, the next branch will probably point to the left due to the substrate removal by the first branch. Such alternation in the branching direction can be seen in many leaves. If the details of a pattern depend on fluctuations but each step has a strong influence on forthcoming decisions, the actual pattern will be unpredictable. Indeed, the venation of two leaves on the same tree are never identical although they are certainly formed under control of the same positional information.
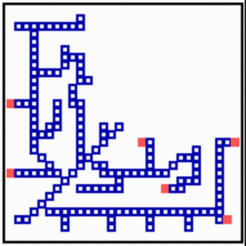
Many net-like structures are able to regulate. New filaments grow in and the defect becomes repaired. Removal of tracheae leads, for instance, to a re-direction and elongation of still functional tracheae into the deprived region. In the model, after removal of some filaments, the substrate is no longer removed and the rising substrate concentrations attract activator maxima from the non-injured region. The regenerated pattern is similar but not identical to the original one, demonstrating that not the final pattern but the mode of generation is controlled in development.
Several factors are known that play a role in the determination of final density of the net. For instance, a tumor can only grow if he is able to attract new blood vessels for its nourishment. In contrast, in cartilage vessels disappear, contributing to the white appearance of that tissue. In the simulation shown below, an increased substrate production in the upper half of the field leads to an increased vessel density. In contrast, an elevated level of constitutive inhibitor production in the center repels ingrowing veins.
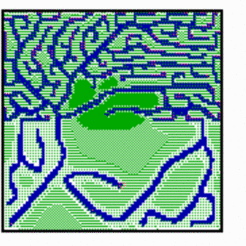
It is a property that networks generated in this way end blindly. This is in agreement with the lymph vessels an tracheae. Other systems form frequently anastomoses. Veins of the leaves in higher plants form such reconnections. The many veins on the wings of dragons flies (below, right) consist on close loops only. Also the early plexus of blood vessels consist on such loops. A possible mechanism to create such pattern is based on the combination of a spot and a stripe system: The spot system dictate where no filaments are formed (Koch and Meinhardt, 1994 ). Therefore, the regions that are free of vessels play an active role. This is in agreement of the shaping of the vessels by growing holes in the plexus [3]
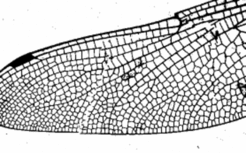
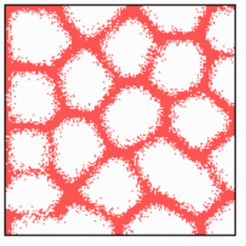
Further Reading and References
Original paper: Meinhardt, H (1976). Morphogenesis of lines and nets. Differentiation*6, 117-123 [PDF]
More details and equations can be found in the book "Models of biological pattern formation", chapter 15; [PDF]
- Shilo, B.Z., Gabay, L., Glazer, L., Reichmanfried, M., Wappner, P., Wilk, R. and Zelzer, E. (1997). Branching morphogenesis in the Drosophila tracheal system. Cold Spring Harbor Symposia on Quantitative Biol. 62, 241-247.
- Hacohen, N., Kramer, S., Sutherland, D., Hiromi, Y. and Krasnow, M.A. (1998). Sprouty encodes a novel antagonist of FGF signaling that patterns apical branching of the Drosophila airways. Cell 92, 253-263.
- Risau, W. and Flamme, I. (1995). Vasculogenesis. Ann. Rev. Cell Dev. Biol., 11, 73-91.